In the study of genetics, linked genes is an important concept to understand genetic sequencing.
This article provides a simple five-step guide to understanding linked genes.
Watch this quick explainer video, then go through each step in this article.
Video:
Summary:
- Thomas Hunt Morgan discovered the first example of linked gene ratios.
- When genes are close together, they cross over and change the probability of parental vs. non-parental phenotypes.
- You can identify linked genes via these ratios and measure how far they are apart on a chromosome.
- Don’t worry if you don’t understand it straight away. Take a break, watch the video again, and repeat the step you understand up to.
Step 1: Parental Vs. Non-parental Offspring
DNA and genetics have a set of rules to reproduce a variety of offspring.
There are two types of traits:
- Parental: Traits in offspring that their parents have.
- Non-parental (recombinant): Traits that offspring have that parents don’t have.
If genes divide equally, there is an even mix of parental and non-parental offspring. In nature, non-parental traits usually occur less often. That’s because of the way linked genes on chromosomes cross over.
When two parents mate to create an offspring, their DNA split during meiosis and multiply. In humans, it’s a crossing of 25 000 genes. That’s roughly 1000 genes per chromosome.
Because there are many genes on one single chromosome, gene transfers occur when chromosomes multiply.
The way that these genes are swapped or ‘recombined’ help parents produce a wide variety of offspring.
This is why linked genes is an essential concept in genetics.
Step 2: Linked genes do not obey Mendel’s Laws of Genetics
The next step is to understand that linked genes are confusing. They are confusing because they do not obey Mendel’s 3rd law of independent assortment.
The law says that is for every gene that code for a trait, genes will be arranged separately.
However, this was true for his pea-pod experiments. In larger chromosomes, where there is more DNA, it doesn’t quite work that way.
Was Mendel wrong? No, he wasn’t.
Genes do assort independently. However, it depends on where they lie on the chromosome.
Linked genes show how genetics becomes more complicated when we look at how cells reproduce.
Genes on different chromosomes will be sorted separately (because they’re far apart).
Genes on the same chromosome, but far apart, will also likely be sorted separately.
Let’s look at the simplest example: genes independently assort
We have four genes:
- Gene A & Gene a (independent)
- Gene B & Gene b (independent)
In a simple Mendelian independent reproduction, the cells are broken in equal 1:1:1:1 split.
AB ab aB Ab – 1:1:1:1
When this happens, offspring will divide into a 25% break down of the different gene splits.
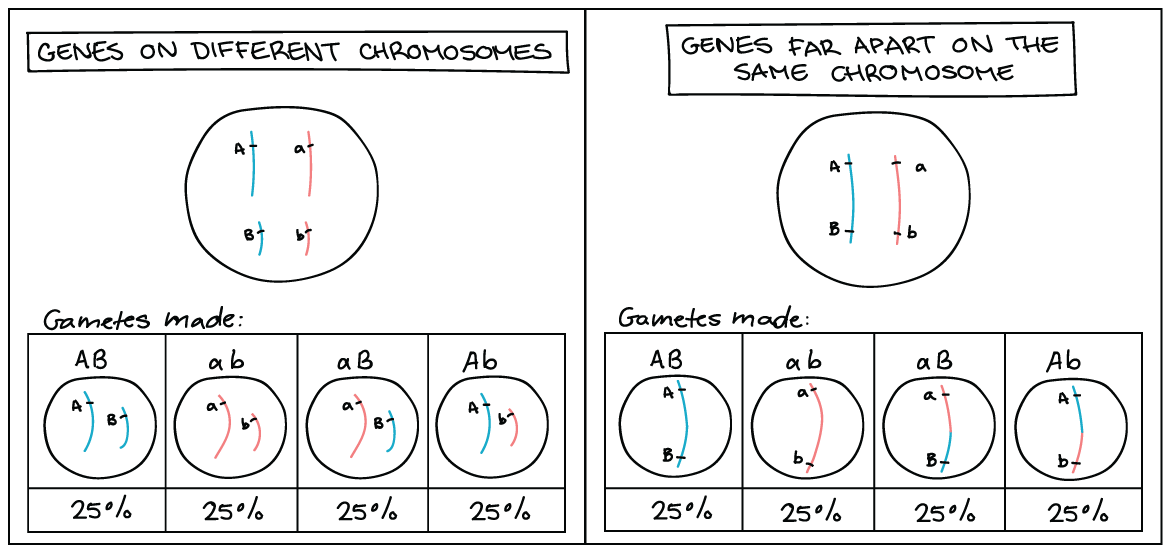
How genes on separate chromosomes in a 1:1:1:1 ratio (law of independent assortment).
In nature, it doesn’t always happen like this. When genes are not on a separate chromosomes (left) or far apart on a chromosome (right), things change.
Step 3: What does it mean for a gene to be linked?
To understand linked genes, we’ll look at a genetic experiment of Thomas Hunt Morgan. In 1908, he studied the eye color and wing size of fruit flies.
[For more background on how he added to pea plant experiments of Mendel, and the chromosome theory of Boveri-Sutton. Read more here]
Morgan’s Experiment
Morgan tested fruit flies with two different traits of eyes color and wing size. These traits were either found normally in nature (wild-type) or mutant traits which he created in his experiments.
Wild-type: Red eyes and large wings.
Mutant type: Purple eyes and small wings.
EYES Gene
Red (wild) pr+
Purple (mutant) pr
Wings Gene
Large (wild) vg+
Small (mutant) vg
He crossed a red eye, large wing fly that carried mutant genes (heterozygous).
Quick Exercise: It will help to draw the above gene combinations (answer below):
Heterozygous wild fly Genes
Red Eyes pr+ pr
Large Wings vg+ vr
Tester Mutant Genes
Purple Eyes pr pr
Small Wings vg vg
The results:
Morgan’s results were different from what he expected.
According to Mendel’s third law, the expected results would be below.
Expected: 50% parental: 50% non-parental (recombinant phenotype)
However, Morgan found the following:
Results: 83% parental 17% non-parental (recombinant phenotype)
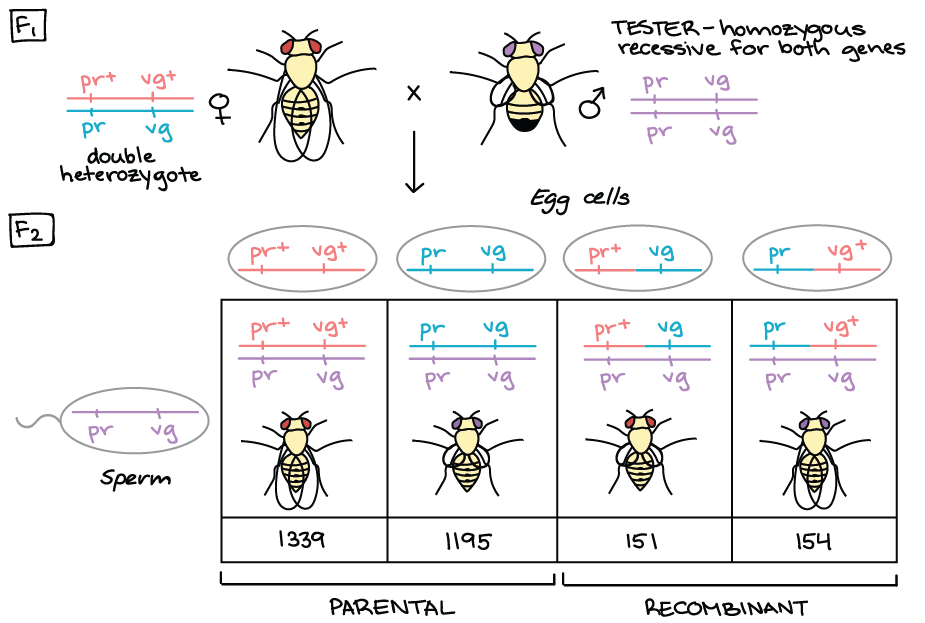
Non-parental (recombinant) phenotype is a child that doesn’t match parents. In this case, they appear in smaller proportions (right) than parental siblings (left). This skewed ratio shows that linked genes crossed over during reproduction.
Morgan found that the non-parental offspring had a much lower ratio (151 & 154).
The experiment had revealed a result that violated Mendel’s law of Independent assortment.
The ratio tells us that the purple and small wing genes are linked.
So how do you know if genes are linked?
Linked genes occur when the ratio of parental vs. non-parental siblings are skewed.
If the four classes of offspring are not produced in equal numbers, there are linked genes.
This was the first evidence of linked genes. Now we need to explain why it happens.
Step 4: What happens to linked genes in DNA during reproduction?
The next step is to explain why the offspring did not appear in a 1:1:1:1 ratio. Morgan had to discover this himself. He realized that there was a crossing over of genes on chromosomes during meiosis.
This was BRILLIANT because NO ONE had put these pieces together before.
Linked genes cross depending on where the two genes sit relative to each other. That may be on the same chromosome or a different chromosome. When they duplicate (meiosis), the chromosomes randomly exchange matching fragments.
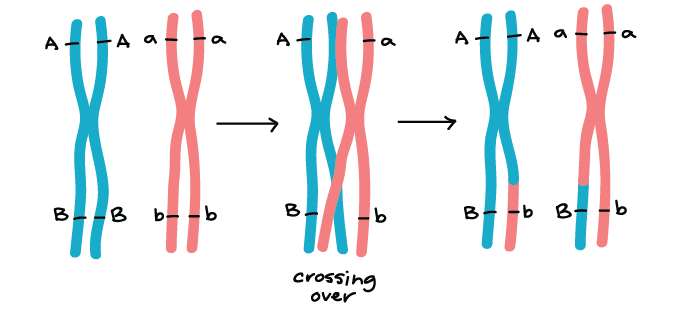
Genes crossing over during meiosis, called homologous recombination.
How does gene recombination happen during meiosis?
As cells reproduce, enzymes cut the chromosome and drag them across to the other chromosome.
The crossover can unlink genes so that you don’t get the same ratio of offspring. The chance of crossing over happening is dependent on how far away the gene is from each other.
Mendel observed that two genes on different chromosomes would have a 50% chance of recombination.
50% represents when:
- Two genes are on separate chromosomes.
- Two genes sit on the same chromosome, but far away.
As genes sit closer together on the SAME chromosome it becomes less likely they will cross-over.
Why is it less likely for genes close on a chromosome to cross over?
Nature has its way of trying to reduce errors when reproducing DNA.
If a cross-over event is happening close together, there is a higher likely-hood of error. Enzymes may create errors when cutting genes close to each other, resulting in DNA error.
The most likely place for a cross-over to happen is further apart on the chromosome.
When genes are far apart, crossing over happens often enough that all types of gametes are produced in the 1:1:1:1 ratio frequency.
Put the genes closer together: and the non-parental (recombinant) ratio drops.
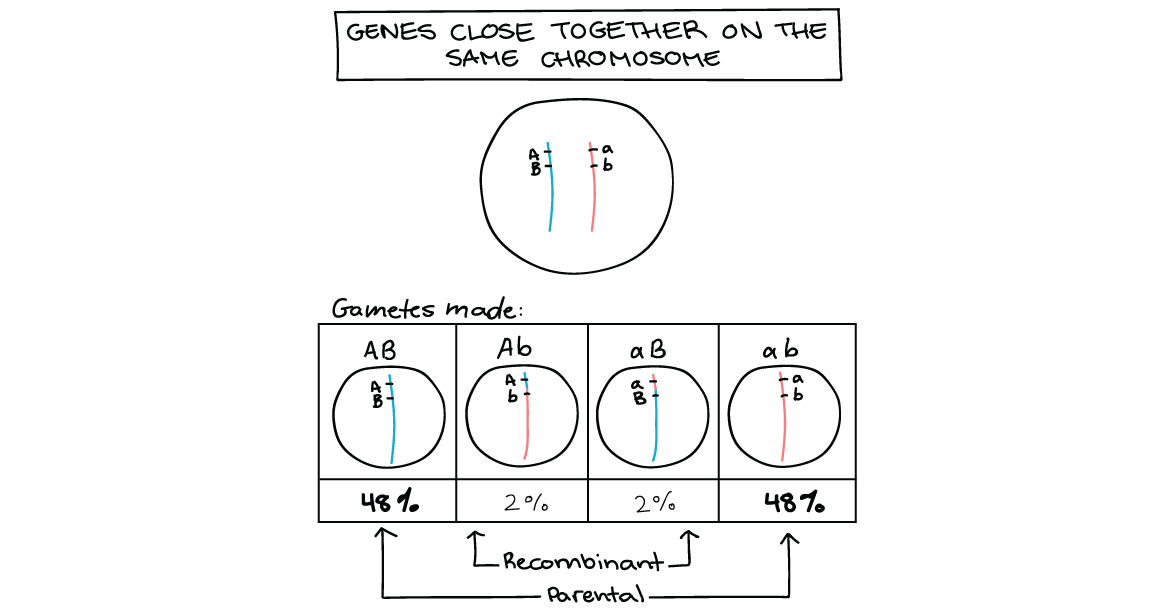
Genes close together on a chromosome have a smaller chance of crossing over due to a higher error rate.
Step 5: Gene linkage, recombination, and gene mapping
The last step is to understand why gene linkage is important in genetics.
Gene linkage helps to know where genes were on a chromosome before gene mapping technology became available.
For example, if you are looking at a combination of offspring, you can compare the parental and non-parental ratios.
You can use these numbers to reveal:
- Which of the genes are linked (non-parental)
- The percentage of non-parental to parental-offspring
- The size of the percentage means the linked genes are closer together
- 1% = 1 gene map unit (explained below)
Let’s look at the fruit fly example again:
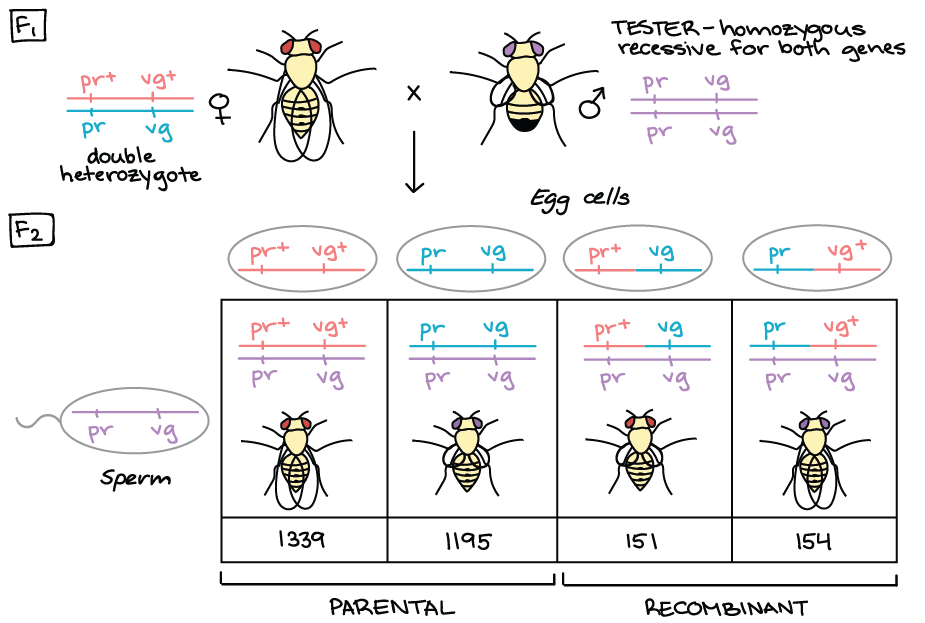
Non-parental (recombinant) phenotype is a child that doesn’t match parents. In this case, they appear in smaller proportions (right) than parental siblings (left). This skewed ratio shows that linked genes crossed over during reproduction.
First: we count the number of non-parental siblings:
Recombination frequency (RF) = Total offspring/Recombinants ×100%
151+154 / 1139+1195+151+154 x 100% = 10.7% of total offspring
REMEMBER the less non-parental offspring, the closer genes.
It doesn’t give us an exact measurement, but a ‘gene unit.’
1% = 1 gene map unit (A map unit represents the total distance within a chromosome).
Now we can estimate that the linked genes are a distance of 10.7% gene units.
If it’s closer, the percentage goes down. (5% non-parental means 5 gene map units distance away)
The final step: recombination frequencies
We now have a gene unit measurement for two linked genes. However, that gives us two random points on a chromosome.
The last step is to use a third gene, look at the ratio between all of them.
Measuring gene map with linked genes
Remember: Recombination frequency “maxes out” at 50% (meaning the genes being unlinked, or assorting independently).
Now, if you add another gene, you can look at recombination frequencies between 3 different genes
For example: A, B, and C, and we want to know their order on the chromosome.
First, we need to know the order: (ABC? ACB? CAB?)
If we look at recombination frequencies among all three possible pairs of genes (AC, AB, BC)

Three genes and their non-parental percentages can be used to map order and distance on a gene.
The largest recombination frequencies mean that the genes are on the outer part of the gene.
That means the order is ABC.
As we add genes (D, E, F, G) the RF distance, shows where the genes are and the order that they are on a chromosome.
Conclusion:
Don’t worry if you can’t understand linked genes on your first time of reading the article. It takes a few times for each concept to solidify in your mind.
If you’re confused, watch the video again, and go back to the step that you understand to continue.
In summary:
- Mendel’s third law does not apply to all DNA chromosome reproduction.
- Linked genes represent a cross-over event when chromosomes create offspring.
- The ratio at which parental vs. non-parental offspring occur show the distance between genes
- The frequency of non-parental (recombination) between genes establishes distance and can be used to build gene maps.
Can you now understand the concept of linked genes and recombination frequencies? Leave your thoughts in the comments below.